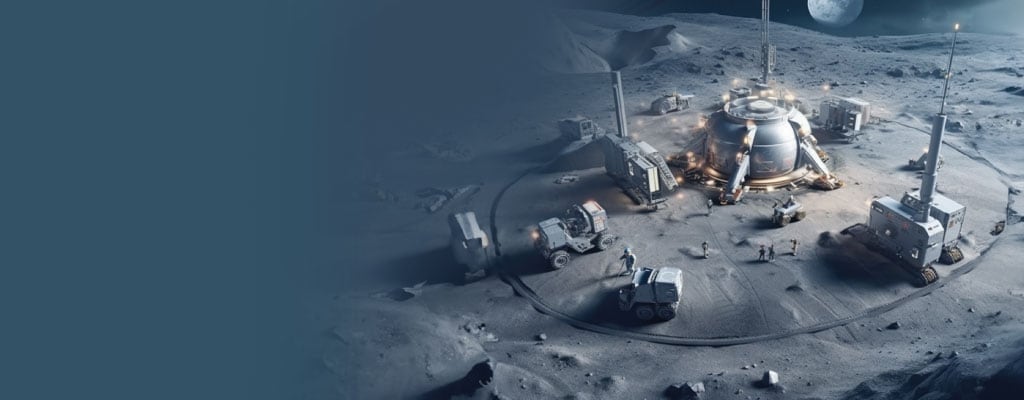
Application
Landers and Rovers Critical to New Moon Missions
Lunar landers and rovers are essential to any future moon missions that will deliver or retrieve people and payload to or from the moon.
Contributors:
- Martin Cullen, Senior, Business Development Manager, Global eVTOL Lead
- Karl Kitts, Senior Engineering Manager, Advanced Systems Architecture
Overview
The moon is often viewed as an untapped, unregulated potential resource for service providers, mining companies, and spacecraft manufacturers. This has sparked a renewed focus in lunar exploration that rivals the Space Race of the 1960s. In the next decade, there will be an estimated 250 new moon missions, including up to 25 missions in just the next few years. In August 2023, India became the fourth country to successfully land on the moon (after the United States, China, and the former Soviet Union) and the first to land on the moon’s south pole.
Such a high frequency of future moon missions will allow for unprecedented opportunities for commercial and scientific exploration. And lunar landers and rovers will be essential to deliver or retrieve people and payload.
Lunar Exploration, Discovery and Solutions
Enabling Lunar Exploration and Discovery
Established aerospace industry titans and well-funded startups are collaborating and competing to stake their claims on the moon and help shape the future. Over the next 10 years, they will develop a wide range of mobile vehicles and stationary lunar landers on the moon.
Landers will be used for scientific experimentation, commercial services, advertising, and promotional activities, and as bases for mining and lunar exploration. Landers will also support rovers with communications by serving as data nodes and providing uplinks to lunar orbit satellites for onward relay to Earth. Rovers will primarily be used to prospect for minerals, transport cargo, crew, and tools, while making it possible to explore, sample, and map the lunar surface.
Missions to the moon will also provide new and exciting opportunities for nations and commercial entities to inspire renewed interest in space and the virtually unlimited opportunities the moon provides.
Purpose-Built Solutions
Currently, each lunar lander or rover is custom designed and built for a particular task. Its size, battery life, and other key specifications are determined by what is absolutely necessary to complete that task.
Some of the first rovers to be deployed will be shoebox- to suitcase-sized devices designed to explore craters and descend into lava tubes to map them, collect samples, search for minerals, or perform water ice detection on the moon. Polar exploration and other major tasks will require larger lunar terrain vehicles with more capabilities.
By the end of the decade, we will begin to see a wider range of rovers, from crude transport vehicles that haul minerals and materials to support helium-3 mining on the moon, to self-sufficient, habitable vehicles able to comfortably carry two or three astronauts without pressurized suits for lunar missions of 30 days or more. Larger vehicles may need to be delivered in pieces, and the lunar rover parts will have to be assembled on the moon’s surface by astronauts or robots to make them operational.
Shift to Standardized Solutions
As the number of flights to the moon increases and the opportunities and demand for lunar landers and rovers grow, manufacturers will begin to offer more standardized designs. Customers will be able to customize the lander, but the basic design will follow a company standard.
This approach is much like what SpaceX has done with its reusable Falcon 9 rocket as well as the fully reusable Starship spacecraft which features a limited set of configurations allowing it to serve multiple roles, such as launcher, crew and cargo transporter, lander, fuel tanker, and more.
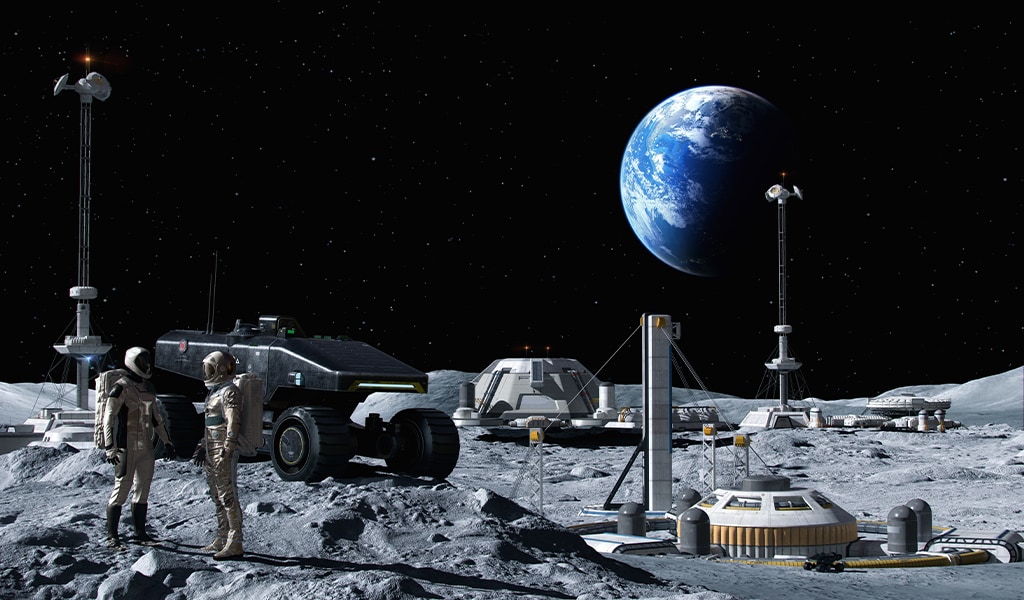
Design Considerations for Lunar Landers and Rovers
When being shipped from the Earth to the moon, landers and rovers are essentially along for the ride as cargo. Therefore, they must be built to survive everything the rocket endures during launch, space flight, and landing, yet be ready to come to life on the lunar surface when commanded.
The following factors must be considered when designing a vehicle that operates on the moon:
- Dust: The moon’s surface poses a major threat to landers and rovers. Its dust sticks to the wheels and underside of the rovers and can damage the vehicle’s critical mechanical and electrical systems. The risk of dust ingression also limits rovers to speeds of 5-10 miles per hour — any faster kicks up too much dust. As a result, vehicles and components bound for the moon must be tested extensively at facilities that are equipped with dust simulators.
- Challenging Terrain: The surface of the moon is full of craters that subject rovers to extreme vibrations and steep angular ascents and descents as they explore.
- Temperature Variation: Temperatures can swing from -180°C to 180°C (-292°F to 356°F) very quickly as the rover moves in and out of shadows of lunar crater.
- Power management: Prior to landing, a lunar lander requires thrust or other functions with high-energy demands. After landing, its power needs become relatively stable and can depend on solar power with a battery back-up, if needed, to survive the long lunar night. However, larger rovers demand more power than solar panels alone can provide. So a battery or nuclear power source is required to support moment-to-moment fluctuations in speed and power needs based on its unique mission. For example, rover tasks like drilling, loading cargo, and exploring require more complex power arrangements than what’s found in a lander.
- Expendability: Smaller lunar rovers tend to be less safety-critical and have a shorter life expectancy as compared to larger rovers. They are also relatively easy to replace. Larger rovers are designed and equipped to conduct moon missions that might last for months so power management, criticality, and durability are key design concerns. Eventually, power stations or electrical charging locations will be available to charge the rover’s batteries as easily as charging an electric vehicle on Earth today.
- Regulations and Restrictions: While there are a number of international treaties and agreements in place, space travel and commerce are not currently subject to a universal set of regulations or restrictions. New laws and regulations are needed for the commercialization of space. In the meantime, each country manages their own space and lunar programs. For example, in the United States, the National Aeronautics and Space Administration (NASA) has authority over government-driven civil activities, while the Federal Aviation Administration (FAA) is responsible for all commercial activities and launches. Regardless of who has authority, civil and commercial missions are all subject to rigid requirements in terms of criticality and reliability.
Critical Decision-Making
Each nation or commercial enterprise must make difficult decisions before committing to a mission. The European Space Agency (ESA) developed a classification scheme to help guide its decision-making process. To rank each ESA mission as Class I, II, III, IV, or V, the following metrics are considered:
- Criticality to Agency Strategy: Includes flagship missions, international cooperation, and the impact on ESA strategic goals and image.
- Moon Mission Objectives: Identifies the mission’s priority and purpose, which can range from in-orbit demonstrations to missions that are purely educational.
- Cost at Completion: Classifies new moon missions by cost, starting with Class V that are less than 1 million euros to Class I missions that cost more than 700 million euros.
- Mission Lifetime: Defines how long the mission will last, from 3 months for Class V missions to more than 10 years for Class I missions.
- Mission Complexity: Considers whether the mission will use new or existing design interfaces, feature unique payloads, or require the development of new technology.
A mission’s class assignment will determine the types of components that are essential to the lander’s or rover’s final design. At the highest level, Class I missions require ruggedized products developed to meet the extreme conditions of space. Less rugged, but still reliable, components may be considered for use in Class V missions.
While this classification system is specific to the ESA, each country or organization must ultimately determine what is acceptable.
New Space Projects
The Growing Impact of Startups
Government organizations and the largest aerospace companies have traditionally been cautious when developing new space projects. Most innovation in lunar lander and rover design is now coming from startups, which are less risk averse and choose to forge new paths. The industry has reached an inflection point where costs are becoming affordable enough that if a startup’s concept appears commercially viable, investors are more willing to take chances on space-based opportunities.
Notably, SpaceX has made access to space much easier and less expensive, which has inspired others to challenge the status quo and take on more commercial risk.
Another example of this new strategy was demonstrated in April 2023 when Japan’s ispace attempted to become the first private company to land on the moon. Despite the initial challenges, ispace is pushing ahead with plans to try again next year in a mission that will include a mix of science experiments and advertising opportunities.
Innovators like ispace and SpaceX are willing to take on more risk and learn from their mistakes, which translates to faster development cycles and lower development costs. Things don’t always go as planned, but this trial-and-error approach may be a more cost-effective and faster way to achieve an end goal.
TE in Space Missions
A Part of History
TE Connectivity (TE) components were used in the first lunar rovers that were deployed in the early 1970s and continue to be used today. TE’s HARTMAN 100 ampere relays (N422DG) and 50 ampere relays (N218DG) have been to the moon. HARTMAN contacts deliver high performance in a ruggedized form that’s a fraction of the size of typical industrial control products used on Earth.
TE’s KILOVAC K40P high-voltage relays have been used on NASA’s Curiosity Rover on Mars and on the BepiColombo spacecraft that’s on its way to Mercury with a target arrival in 2025. KILOVAC relays are also used throughout the International Space Station and continue to be used extensively in space satellite programs.
Additionally, TE’s KILOVAC CAP120 high-voltage contactors, ESA qualified SPEC 55 wires, DBAS connectors, RF connectors, and MICRODOT coaxial connectors are in use on India's Chandrayaan-3 mission which landed on the moon in September 2023.
Future Moon Missions
Ready for the Future
At TE, our products are only one part of the solution. We also have the experience and capabilities to adapt existing products from other industries or applications to help meet the extreme demands of the lunar environment. If existing components originally designed for use on Earth aren’t able to withstand rigorous space simulation testing, TE engineers can work to help develop new, more robust solutions that can.
Summary
Key Takeaways
- An increasing number of countries and commercial organizations are racing to be the first to capitalize on the untapped potential in resources and opportunities the moon provides.
- Lunar landers and rovers are essential to any mission that will deliver or retrieve people and payload to or from the surface of the moon.
- Today, each lunar lander or rover is designed and built for a particular task. However, a shift to more standardization is on the horizon.
- Each nation or commercial enterprise must consider environmental and criticality factors before committing to a lunar mission.
- Most innovation in lunar lander and rover design is coming from startups that are less risk averse than traditional aerospace leaders.
- TE Connectivity offers the products, experience, and capabilities that enable landers and rovers to meet the extreme demands of the lunar environment.