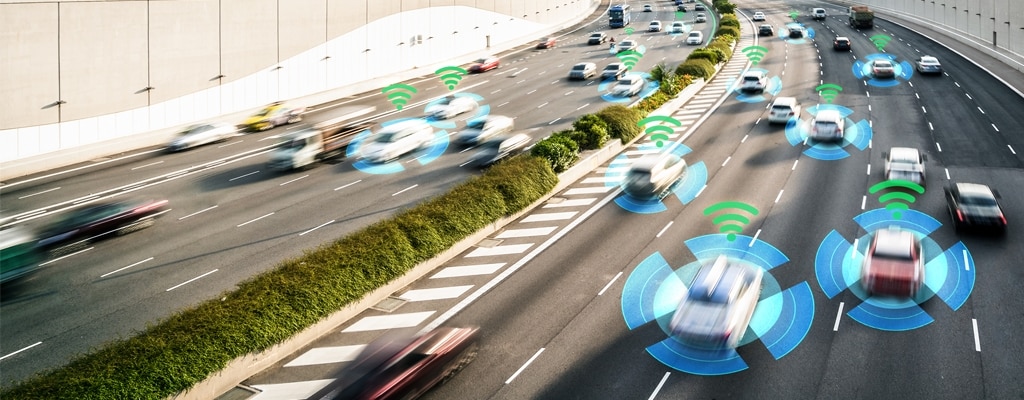
Application
LiDAR Sensors for Automotive Safety
A LiDAR designer’s guide to sensor technologies for automotive/mobility systems

Today’s LiDAR systems are finding new uses from security to mapping to industrial automation. One sector with particularly high levels of interest and development: the mobility market. LiDAR scanners are critical components in prototype systems for autonomous vehicles, as well as in current systems for adaptive cruise control (ACC), collision avoidance systems, traffic sign recognition, blind spot detection, and lane departure warning. None of these LiDAR-based systems can function without a key component: their sensors — the “eyes” of the system.
Background
Mobility light detection and ranging (LiDAR) systems must sense the environment quickly and reliably, assembling as detailed a picture as economically feasible of immediate surroundings and the road ahead. Far ahead: systems mounted in fast-driving cars need to “see” a minimum of 150 meters (almost 500 feet) forward, and detect small objects down to 10 centimeters (about 4 inches) in height.
All this presents challenging technical requirements for the systems’ sensors.
The mission demands complementary but independent sensor systems, with guaranteed functional safety and environmental qualification. For example, units should be rated for operating temperatures from -40 to 125 °C (-40 to 257 °F) to accommodate both environmental heating and heat from other system components. Sensors must possess an optimum signal-to-noise ratio, to “see” the signal through any distracting background. And since optical detectors must be prepared to deal with varying levels of environmental light, sensors should possess a wide dynamic range.
(Note that the term “detector” may refer only to the photoelectric detecting element, while “sensor” includes the detector plus adjacent electronics that provide functions such as connectivity. The terms are sometimes used interchangeably.)
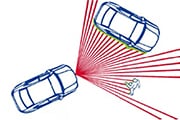
LIDAR in Action
Besides basic physics, LiDAR system designers must also consider basic economics. All components in the car should be maximally cost-efficient. For practical purposes, the best cost/performance ratio trumps the best technology. All current automotive mobility systems using long-range LiDAR are “scanning” devices, that move the laser beam step by step over the whole scene. Effective range using current technology: 30 to 300 m (about 100 to 1000 ft). Almost all are built around 905 nanometer (nm) lasers. These emit invisible beams, are available at low cost in high volumes, and use high power for short pulses (for example, 75 W peak for 5 nanoseconds) — presenting an optimum power-to-cost ratio. These lasers have been extensively used with mature, inexpensive silicon detector technology.
Selecting the Best Sensor Technology
As the industry moves forward, design engineers are applying several different sensor technologies for LiDAR mobility systems. Each has advantages and drawbacks, as described below.
Silicon PIN diode detectors
These silicon-based detectors possess a structure featuring three semiconductor types layered together: P-type / Intrinsic / N-type.
They exhibit good dynamic range, with the ability to handle widely varying amounts of light. For example, they can detect the reflection of a distant object, even when subjected to direct sunlight. And they are relatively inexpensive.
However, they cannot deliver the high levels of bandwidth or signal-to-noise performance most modern mobility LiDAR systems require. Finally, they are neither very sensitive, nor very fast.
Silicon photomultiplier (SiPM) and single-photon avalanche diode (SPAD) detectors
Their makers originally developed these solid-state, silicon-based sensors for small, specialized scientific and medical applications. More recently, they have attempted to try them out in the larger LiDAR market.
These sensors function similarly to APDs (see below), but are optimized for very high internal amplification or gain, making them able to detect the smallest amounts of light. They are also very fast. Finally, they are compatible with commonly available CMOS technology, and so can be paired with associated electronics on the same chip.
However, the sensitivity of their single-photon counters is much lower than that of APDs. So they must rely on very high multiplication. Unfortunately, the multiplication process adds noise that often significantly degrades the signal/noise ratio. Their amplification mechanism is also prone to false triggers caused by high temperatures. Perhaps these sensors’ most serious drawback: their high gain comes at the cost of saturation problems.
To begin with, the sensors must deal with laser light reflected from objects ahead. In addition, many LiDAR systems specify scanners with wide fields of view. This places quite a large amount of added light on an SIPM or SPAD sensor. And some phenomena routinely encountered in LiDAR mobility environments — such as bright sunlight, high-beam headlights, or other LiDAR systems — can saturate the sensor with higher light levels than it can handle, even when using optical filters. As development work to offset their drawbacks continues, these sensors are often considered for various LiDAR applications. But to date, their saturation issues and other problems mentioned above keep them from becoming the detectors of choice for scanning long-range LiDAR.
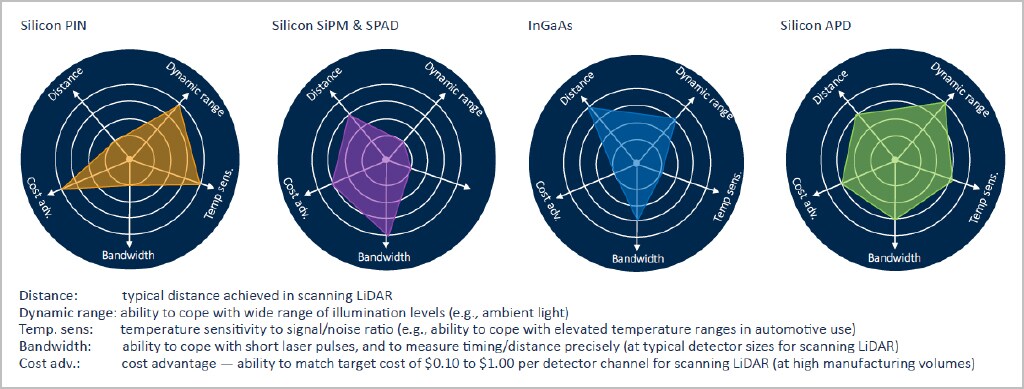
Figure 2 Comparison of detection technologies
Indium Gallium Arsenide (InGaAs) Photodiode Detectors
These sensors are frequently utilized at small sizes in telecommunications glass fibers, but are newcomers to LiDAR, except for specialized military or aerospace applications. This technology abandons conventional silicon-based construction for InGaAs material.
With laser systems specially built for its higher spectrum (1550 nm, versus 905 nm for the other sensors discussed here), this design should be more sensitive, and able to put out more power. Thus, it can enable an automotive LiDAR system with a longer range than most other sensors.
However, InGaAs detector performance can be significantly degraded by even slightly higher than normal ambient temperatures. The sensor may well need an external cooling system, even in temperate climates.
In addition, its base material is significantly more expensive than widely used silicon substrates. And fabricating InGaAs sensors in large sizes for LiDAR use would require much more complex manufacturing than silicon designs. To date, they have not been successfully made in high commercial volumes.
Finally, since this technology is new to the automotive LiDAR world, OEMs would have to be prepared to spend substantial time, effort, and revenue trying to develop a new LiDAR system around any InGaAs detector.
Avalanche Photodiode (APD) Detectors
Originally perfected for industrial and military applications, these silicon-based photodetectors work by enabling incoming photons to trigger a charge avalanche, multiplying gain by their internal amplification mechanism. Their absorption-optimized structure converts at least 80% of a laser’s reflected 905 nm light into photoelectric current. Result: greatly increased sensitivity.
Besides their notable sensitivity, APDs have an optimal signal-to-noise ratio, minimal saturation, and very good speed. They are also among the lowest-cost sensor technologies available.
A potential drawback: APDs use specialized bipolar technology not compatible with commonplace CMOS fabrication. So they can be sourced only from a small number of suppliers. And they cannot be paired on the same chip with their associated CMOS electronics.
However, experienced suppliers can fabricate packages with sensor and electronics on closely adjacent chips. Both can be optimized for best-in-class performance, without compromises. For example, an APD sensor array can be complemented by specially designed transimpedance amplifiers (TIAs) — with customized gains and bandwidths — to convert the photocurrent to voltage, and to condition the signal going into the system for high gain. This can maximize performance, especially in low-light conditions.
APDs are produced by well-established, high-productivity commercial manufacturing processes, and are proven in a wide variety of systems already on the road.
Basically, when done right, they combine proven performance with an attractive price. Currently the detectors of choice for automotive long-range LiDAR, APDs are critical components in a number of today’s most advanced mobility systems.
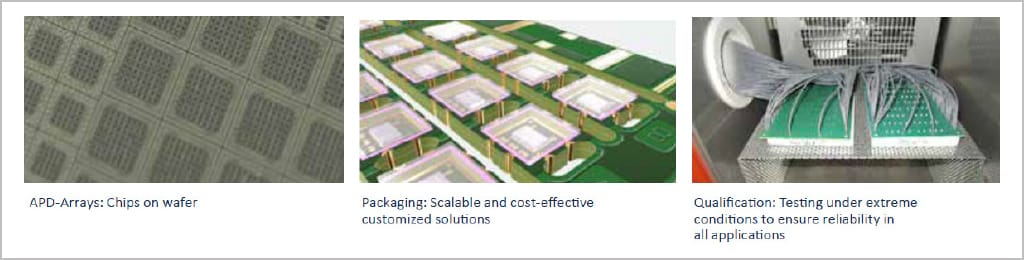
Figure 3 Avalanche photodiode detectors (APD)
Selecting the Best Sensor Supplier
Once the right sensor technology is determined, LiDAR system designers still face the challenge of choosing the right sensor supplier.
Candidates must be evaluated carefully. Do they have the technology, capacity, and know-how to adapt their sensors and systems to an OEM’s individual requirements and markets? Will they work closely with the OEM team on design, manufacture, and scheduling to ensure a winning time to market?
Insist on Experience
If a sensor vendor must spend time bringing its development, manufacturing, automotive qualification, and other processes up to speed, the LiDAR mobility OEM will lose the race for the fastest time to market.
Sensor suppliers gain the experience by doing the work. A good candidate will have already applied their sensor/detector technology for mobility applications. This might include standard and customized APD design; standard and customized dies, packages, and modules engineering and manufacture; and best-in-class electronics.
An ideal supplier will have a proven track record, with products such as automotive-grade APDs and related electronics already in use by major LiDAR OEMs.
Assess for Integrated Manufacturing
Designers should prioritize a supplier with relevant technical advantages, such as lowest noise and highest sensitivity. But they should also look for a sensor maker that maintains holistic control of its domain.
The entire production process should be implemented as a coherent whole — from processing the chips through to prefabricating sensor systems. By manufacturing all central components in-house, a supplier ensures the long availability of all OEM products for series production and aftermarkets.
Check Customization Capabilities
To be successful as a LiDAR system maker, it’s important to achieve the best cost/performance ratio; that can help differentiate a given system from the rest of the overcrowded market. Off-the-shelf sensors may well not fit the bill. Instead, components must often be customized to precisely suit a chosen system design.
System makers need to find a sensor supplier that’s agile and responsive. In many cases, a supplier must work with OEM designers to customize the sensor and related electronics for the tightest possible integration with the rest of the system — and, thus, for optimum performance.
Examples: the team needs to establish sensor geometries that fit a given choice of lenses; to optimize dimensions; and to otherwise adapt to the configurations of each unique optical design. The team must determine the optimum channel count — how many signals are received in parallel — to maximize the spatial resolution of the scanner. And it must customize packaging for the shortest possible interfaces between sensor and electronics.
Finally, a superior supplier should provide sensors possessing refined technical advantages such as multi-pixel homogeneity. If photodiodes are not homogeneous and/or are diversely sourced, they will react differently to ambient temperatures under real-world use. This can significantly degrade LiDAR scanner performance. By contrast, multi-pixel homogeneity can provide the tightest possible signal information distribution, even at peak distances.
Selecting the Best Sensor Supplier
An outstanding sensor supplier should already know the “rules of the road.” It should be experienced with the latest automotive qualification, robustness validation, and characterizationstandards and regulations.
Examples include ISO/TS 16949 automotive-certified production and testing, and AEC-Q 102 and 104 automotive-qualified APD array packaging. The supplier should be able to apply these and other relevant standards to all its components and manufacturing facilities, to comply with regulations and to help system OEMs avoid liability.
Increasing regulation is inevitable. Suppliers should show demonstrated compliance via documented best practices, such as the rigorous self-qualification pioneered by Ford Motor Company in its Q Program.
Look for Future-Proof Support
A supplier should also demonstrate a proven track record of quality and delivery performance, as well as strong levels of support, from initial development through to maintenance service.
Sensor design should be factored in from the start of system design. The earlier an OEM involves the sensor supplier, the faster and easier the entire design/manufacturing process becomes — and the better the resulting LiDAR system performs.
Finally, a supplier should always be looking ahead to future developments in this fast-changing field. The right sensor maker will have an innovation roadmap of expected regulatory, business, and technological developments to come, to help system makers navigate this fast-changing market.

CONCLUSION
The sensor is the eye of every LiDAR system. System designers can choose from several competing sensor technologies. Many design engineers feel that APD sensors have proven to offer the best combination of performance and price. LiDAR system makers should also consider a number of factors in choosing their sensor supplier — including experience, customization capabilities, and automotive qualification expertise. As LiDAR and other mobility technologies continue to evolve, making the right sensor choices clearly marks the path ahead.
Authors:
Dr. Marc Schillgalies, TE Connectivity
Paul Sharman, TE Connectivity