TE engineers discuss vehicle-side thermal equation in relation to DC fast charging within electric vehicles.
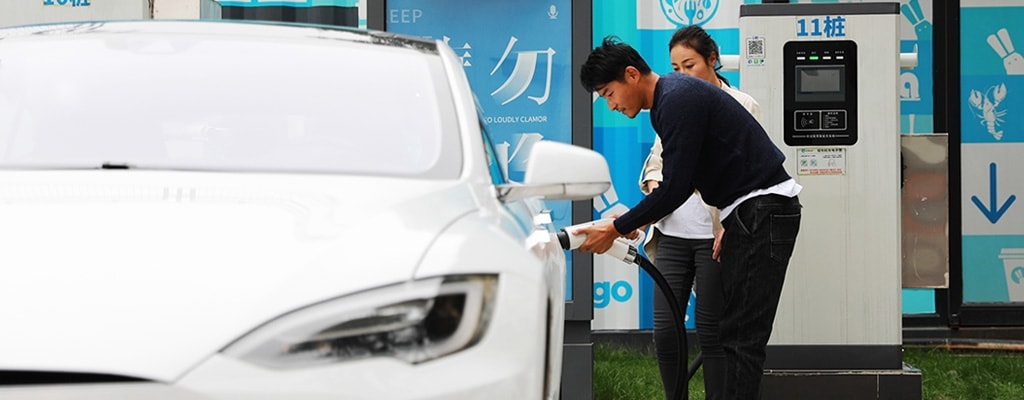
Innovation in Electrified Mobility
The next-generation of EV technologies
EV engineers rely on interconnection, switching, and sensor technologies to advance low-voltage data connectivity networks and high-voltage drive systems. To achieve this, these technologies must work in parallel, within increasingly integrated centralized vehicle architectures.
It is often said that the next generation of mobility - offering safer, greener more connected means of transport, will be characterized by vehicles running on two types of fuel: electricity and data. However, while safety, convenience and the desire for ubiquitous connectivity are driving an ever-increasing number of data-dependent cloud/sensor-based applications within vehicles, many consumers, despite their environmental concerns, are still somewhat reluctant to fully embrace electro-mobility technology.
..”all electric conductive components…..in the complete electrical system, connecting the charging point to the vehicle battery, must be designed and dimensioned mechanically, electrically and thermally.”
Driver Concerns
Major concerns for many potential electric vehicle (EV) drivers are range and charging convenience - in terms of time-to-charge and the availability of charging points. In this regard, connectivity and the connector components from the charging inlet, through the EV battery to the electric motor play a vital role in the safe and reliable high-power fast charging of the additional range that would help allay consumer concerns.
Today charging a vehicle at the direct current (DC) fast charging points, usually found on highway fuel stations, can take up to an hour, depending on the charging power and battery capacity, to add around 300km of driving range. However, the industry’s goal is to add the same amount of range in around 10 minutes, which is comparable to the length or a refueling stop for an internal combustion engine (ICE) vehicle.
Connectivity Challenges
This high-power charging (HPC) can involve 350kW of DC charging power at currents of up to 500 amps, which represents the highest load state for the entire electrical system in an EV. Even hard acceleration, when the driver demands lots of power, does not result in the same magnitude of average current.
This level of continuous current causes high levels of heat loss due to the inherent electric resistance of all components (connectors, cables) within the EV powertrain and is further exasperated when the vehicle is not moving and there is an absence of natural convective cooling.
Therefore, in order to avoid over-loading / over-heating, passive or active heat dissipation or the ability to facilitate a controlled charging current de-rating, are key requirements of the design and dimensioning of all electric conductive components. This means the complete electrical system, connecting the charging point to the vehicle battery, must be designed and dimensioned mechanically, electrically, and thermally.
A major challenge is that the higher the current, the larger the required cable cross-section to carry the power at the same level of voltage without over-heating. Within the vehicle, this is primarily a matter of weight and available space.
For example, there is a considerable difference, in terms of cost, weight and bulk, as to whether a 50 mm2 cross section or a 95 mm2 cross section conductor between the inlet and the battery will suffice. If HPC is to be a realistic proposition, the over-dimensioning of the cable and all the other electrical components must be avoided.
To date electrical component design has not really reflected the dynamically changing current load profiles between high peaks and lows of driving or the requirements of HPC.
Existing standards are based on static load points originally used for the design of relays and (switch) fuses, which are determined by statistical methods reflecting the frequency at which they occur and their importance. This leads to current load values representing static conditions, with a corresponding electrical interconnection component design which does not reflect reality - often including safety margins of up to about 20 per cent.
Our portfolio of connectivity solutions for hybrid and electric vehicles cover the entire range of electric powertrains, providing a complete line of connectors, headers, relays, contactors, harnesses, and disconnects to safely connect and protect the power flow. Our technologies leverage decades of experience with high-voltage generation, transmission, and distribution, in four application areas: HV auxiliary modules, HV powertrains, battery connectivity, and charging inlets.
Battery Connectivity
To facilitate a peak load of 350 kW charging power and beyond, requires a different approach to designing the electrical components. At TE, we are developing a new methodology which dynamically determines the temperature increase caused by components and the heat dissipation in the system at all times.
Based on systemic and near real-world thermal simulation, it makes it possible to examine the component design earlier and predict its performance during various operation modes throughout its entire lifespan. This facilitates safe long-term operation as well as a design that enables improved usability. Model-based thermal simulation provides a verifiable basis for future load profiles which facilitate a proof of safety, reliability and durability of all interconnection components along the high voltage / high current path.
In the same way that the internal combustion engine (ICE) was considered the heart of the traditional motor vehicle, the battery is considered the heart of electric vehicles and many of the same design challenges apply.
Battery electric vehicles (BEV) require more power for the power train. This challenges the integration, into the physical layer, of the automotive board net, sometimes contradicting requirements.
Engineering the next generation EV powertrain connectivity brings with charging inlets, high voltage connectors, relays and contactors as well as miniaturized low voltage connectors for EV battery management systems.
Learn about the options for high-speed connections with automotive-typical channels, taking environmental influences into account, with practical examples showing the urgency of channel analyses using simulation tools.
During all vehicle operation modes, power must flow safely in and out of the battery. Therefore, the main battery connection, and in certain cases, the connections between cell modules, must be able to carry currents up to 600 Amps for several minutes during a high-power charge cycle and significantly higher peak levels during hard acceleration.
Battery module connections also constitute the physical layer for battery protection (over-heating shut-down), battery management (charge states, e.g. state-of-charge SoC) and cell-balancing (charge voltage equalization between cells with different SoC levels). To ensure these operations, every individual battery module must feature failproof electrical connections.
This requires highly integrated contact systems, which must not only be capable of supporting full functionality over the vehicle’s complete lifespan (e.g. 300,000 km / 10 years) but are also robust enough to prevent vibration and temperature stress adversely affecting the contacts’ mechanical and electric properties. In addition, contacts must also be fully touch-safe in order to eliminate potential high-voltage and high-power hazards to ensure safety towards manufacturers and service personnel.
By way of example, a primary design objective, of TE’s BCON + module connector system was to handle constant currents of 400 Amps (with short peaks of up to 1,200 Amps) and voltages of up to 1,000 V, and to operate reliably at typical battery ambient temperatures from -40° C to +80° C. The main challenge was to minimize contact resistance at each contact point to ensure the least possible power losses and heat stress as well as achieve life-time reliability through high vibration resistance according global OEM specifications.
Battery Management
A battery management system is the electronic system that manages the battery pack’s charging and discharging of the cells within. It protects the battery from operating outside its safe limits by monitoring temperature and voltage signals from the cell modules as well as pack-level current signals and transmitting them to the elements that balance or control the cell module environment.
The battery management system is critical to the optimum performance and safety of the battery which means it must operate with a high degree of accuracy and reliability and must be of highly robust construction. However, it must also be compact and lightweight, adding the least possible bulk to the overall battery pack geometry.
Connectivity is also a key enabler of battery management. It is required to transfer signals to the Battery Management Controller (BMC), where they are processed and sent to the Cell Management Controllers (CMC) to balance the cells and enable a controlled flow of power, for example during charging.
BMS electronics require highly compact, yet flexible connector systems. Given that the ratio between battery cells and cell controllers vary according to battery design requirements, such as capacity and vehicle energy demands, connector systems must have the flexibility to accommodate multiple connector configuration permutations. Connectors should also have the flexibility to support different types of cables, including FFC/FPC (flat flexible/ printed cables) that can be routed around compact and complex battery geometries.
In addition, the connector system requires a safe “creepage” and clearance distance between the pins, ensuring no risk of failure from short circuits caused by dust pollution or arcing. As battery modules are supplied as sealed components, manufacturers must be assured that all the internal connectors meet the strict specifications for automotive grade robustness and reliability.
Understanding connectivity solutions for the next generation of mobility. The physical power and data network layers, comprising cables and connectors, will play a pivotal role as the backbone of next-generation safer, greener connected vehicles that consumers want to own, drive or be driven in.
This means low voltage data connectivity networks and high voltage (HV) drive systems must work in parallel, ultra-reliably and safely in increasingly integrated centralized (“smart”) architectures.
Interconnection, switching and sensor technologies will all be key enablers of the innovations that will make this a reality. With its extensive technology-leading portfolio and expertise in these areas, we are in an ideal position to work with automotive technology companies to co-create the connectivity solutions for the next generation of mobility.
Authors
- Qiong Sun, Vice President, Global Automotive, Hybrid & Electric Mobility Solutions
- Uwe Hauck, Director, Product Management, Automotive, Hybrid & Electric Mobility Solutions
- Luis Puras Trueba, Senior Manager, Product Management, Automotive, Hybrid & Electric Mobility Solutions
- Chris Yeadon, Senior Manager, Product Marketing, Automotive, Hybrid & Electric Mobility Solutions