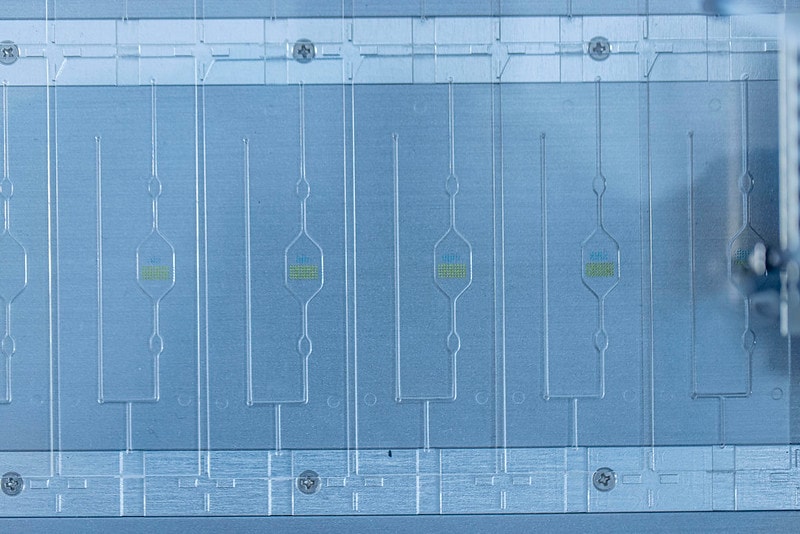
How Assays Impact Microfluidic Design: Reagent Considerations
The first component to be considered when designing a microfluidic system is the device itself.
It may not be obvious, but the device material can chemically interact with the other reagents in a manner that can be harmful to the assay. For example, a device may absorb one of the critical reagents over time and render it unavailable to be used in the assay, or leach chemicals that interfere with the assay. Conversely, some of the reagents used in assays can attack the device itself: some chemiluminescent-triggering reagents contain oxidizing agents such as peroxides and nitric acid and autofluorescence is the bane of any fluorescence detection system.
Device Fabricators
Choose your device fabricator carefully. It’s one thing to injection-mold billions of electric switch plate covers, but it’s another to produce millions of devices with consistent dimensional and chemical properties. Work closely with the fabricator to ensure that he understands that the same raw materials must be used in his process over time and cannot be changed without ample prior notice and testing of the functionality of the altered device.
One injection molder we worked with decided to save money by reusing some scrap plastic from prior runs in molding new parts. The new parts looked the same, but antibodies would not coat on them.
At another molder, a college student who was working for the summer decided to speed up the molding cycle time by spraying a commercial non-stick frying product on the mold. It sped up the molding process, but the parts were unusable.
Critical Reagents in Assays
Most assays use two critical reagents: one reagent captures the analyte of interest, and the other reagent produces a detectable signal from only those captured analytes. These reagents are constructed from three types of base molecules: antibodies, probes, and aptamers. For example, in a sandwich ELISA, the primary and secondary antibodies target different epitope or regions of the analyte to capture the target of interest and then detect as subsequent steps with sufficient washing between each step. In molecular assays, these would be probes to different regions of the target nucleic acid.
Molecular assay probes are synthesized. This provides significant consistency and cost advantages. However, this is not the case with antibodies. Here, the starting points are usually animal species which have specific phylogenetic properties which include rabbits, mice and rats, horses, goats, sheep, and even camels. Campaigns to raise antibodies take six months to a year before they can be screened for use. Since many antibodies are commercially available off the shelf (OTS), using them can shorten the timeline. The tradeoff is that OTS antibodies are more expensive than made-to-order antibodies and multiple vendors should be evaluated for test specificity and sensitivity. Additionally, since OTS antibodies are provided for multiple customers, vendors can change processes as needed and without notification. A continuous supply of test-specific antibodies can be improved by requiring the antibody supplier to be certified (FDA, GMP, GLP) and accredited (ISO, EMA, ICH) or audited. Some catalog antibodies have proven to be of questionable origin and asking the “audit question” can uncover this unreliable source of a critical raw material.
Once candidate reagents have been identified, preliminary screening for desired properties of sensitivity and cross reactivity can be done on a platform (such as a microtiter plate) other than the microfluidic device under development. However, it is mandatory to do the final testing on the device itself. Antibodies that worked perfectly well on one platform may not function as well on another platform for reasons that are still not understood.
Capture reagents are usually immobilized in the device to facilitate separation of bound and unreacted analyte and detection reagents. Washing the bound components enhances sensitivity and reduces non-specific binding.
Know about our expertise in microfluidic development
Coatings
IVD surface coatings are thin layers that are cured and/or dried to change the functionality of a surface. These coatings can be passive (where there is no pretreatment of the surface to be coated) or they can be active (where the surface is chemically modified to encourage binding of the capture reagent). There are thousands of different types of coatings, all dependent on the functional goal of the device: surface tension or wettability, binding of a molecule or side group, environmental or chemical protection, achieving optical properties, or others like defoamers, fungicides and bactericides. Examples include silanes (the most common, and have been used to derivatize glass, silicon, and plastics), perfluorocarbons (excellent for hydrophobic surfaces), and glutaraldehyde (or another bifunctional reagent). These can then be used to couple probes or antibodies to the surface.
Induced magnetic particles have also been used as surfaces for immobilization. One big advantage is that they can be coated in a batch process and then added to the device. Magnetic particles are predominantly used for an on-device purification process: in the absence of an external magnetic field, the coated particles can react efficiently with the analyte and, after application of a magnetic field, can purify the captured analyte by washing away any unbound material. This would be as if the capture reagents had been immobilized on the device.
Detection Chemistries
Detection chemistry is the heart of the diagnostic test. It is a research area that is constantly changing. Every year, new companies introduce new technologies and new detection chemistries to measure ever smaller amounts of analyte. Any viable detection chemistry must have the critical requirements of sensitivity, specificity, and linearity; in other words, a reliable and consistent response that is proportional to the amount of target analyte over its clinical range.
Spectrophotometry relies on measuring the absorption or reflectance of light shone through a chamber on the device and the detection reagent (or reagents). The challenge for this detection mode is that microfluidic devices can have short light absorption pathlengths of sub-millimeters or require unique chamber orientations to increase the readable pathlengths. One example that used short pathlengths relied on a proprietary process involving a gold-labeled “antibody” as the detection reagent and adding a reducing silver salt solution to generate crystals of silver metal that absorb light efficiently.
Fluorescence is another optical detection method where incident light is used to excite fluorescent molecules covalently attached to the detection reagents. The detection reagent becomes excited by the incident light wavelength and emits light at a longer wavelength. The emitted light is directly proportional to the amount of analyte captured, but the excitation/emission wavelength and emission intensity are fluorophore dependent. Typically, bandpass filters are required to ensure only the emission wavelength reaches the CMOS sensor and not the excitation wavelengths and auto-fluorescence.
Electronic and electrochemical detection relies on measuring the generation or consumption of electrons. This is the most common method for glucose monitoring. Electrochemical detection has also been used, but it requires circuitry that must be manufactured into the device. The method also places some additional constraints on reagent purity to be viable
Chemiluminescence is one of the most popular choices for detection chemistry because it is extremely sensitive. While there are two basic types of chemiluminescence (glow and flash), glow chemiluminescence dominates the IVD industry. Glow chemiluminescence relies on an enzyme-coupled detection reagent that acts on a substrate to generate an unstable intermediate that gradually decays with the emission of light. The assay requires the addition of a separate reagent (or reagents) to the assay to generate the light: the trigger reagent is a mixture of a peroxide and luminol that must be mixed just prior to contact with the acridinium ester as the triggering mixture is unstable. Chemiluminescence approaches come at a cost of greater fluidics complexity over fluorescence to achieve enhanced sensitivity.
Sample Processing Reagents
Capture and detection reagents could not function in the absence of a range of other regents. Coating buffers, assay buffers, sample preparation buffers, and neutralization and wash buffers play critical roles in making the assay functional. Adding compounds that ensure the pH remain in fixed ranges is a common practice. Other salts, added to maintain the ionic strength at fixed levels, help keep proteins folded in their natural states. Tightly controlled salt concentrations are mandatory in molecular assays to keep probe and target hybrid pairs stable. Both are negatively charged and would repel each other in the absence of high salt concentrations.
Detergents are included to improve surface wettability and flow characteristics, but care must be taken to keep a minimum of surface tension for efficient operation of fluid control in the microfluidic flows, especially for active or controlled fluidics. Some development scientists have a fascination with expensive exotic buffers that seldom perform any better than ordinary phosphate buffered saline (PBS) solutions. Managers would do well to ask the question, “Is this the only buffer that can accomplish the needed requirement?” Buffer simplification and standardization saves money.
Buffer Storage and Stability
For point-of-care and over-the-counter products, buffers are stored onboard the microfluidic device and disposed of after use. Reagents need to be stable for at least six months to be commercially viable, and that shelf life is typically extended to a year with additional stability data. Microorganisms are ubiquitous in the environment; in the absence of preservatives, they will happily consume these reagents.
Cocktails of preservatives may be needed to control both bacteria and fungi like yeasts and molds. Sodium azide is an effective and inexpensive bacteriostat. Freeze drying either in the microfluidic device or as a tablet to be added to the device can also be used to preserve reagents. To keep freeze dried reagents in their desired state prior to rehydration, care must be taken to exclude moisture from the device. Plastic films used to seal microfluidic trenches into tunnels are surprisingly porous to water vapor so Mylar bags with desiccants may be necessary. Light and oxygen can also damage reagents and care should be taken to exclude them.
Reagent stability must be demonstrated with rigorously conducted stability studies. Real-time studies can be supplemented with accelerated studies that can be used to predict shelf life. Elevated temperatures are employed to accelerate the reactions that cause reagent deterioration. Literature exists to help extrapolate reagent stability due to shipping and storage requirements with upwards of 50 degrees C, and shelf requirements at “room temperature” or in a fridge at 4 degrees C. Note that some regulatory jurisdictions (such as Japan) will not accept accelerated stability studies and insist on real-time data.