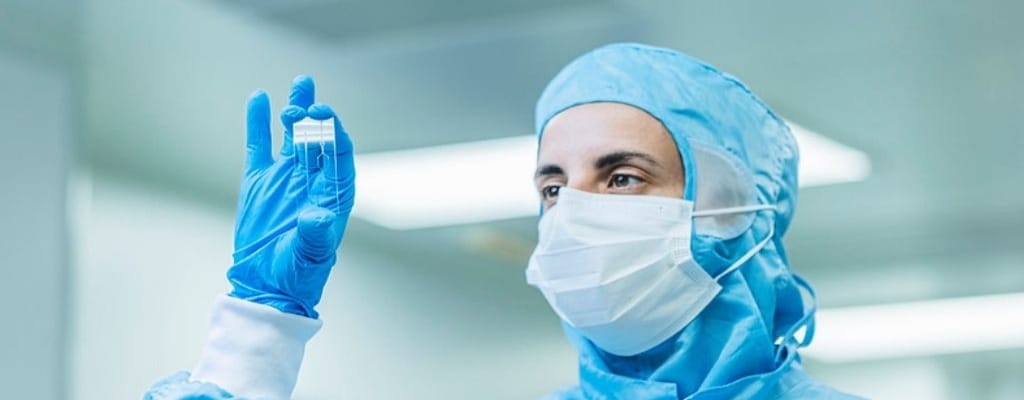
Designing a Microfluidic Bioanalytic Device? Factors to Consider…
Microfluidic devices (and their cousins, nanofluidic and mesofluidic devices) are increasingly used in an impressive array of bioanalytical applications. These range from familiar in vitro medical diagnostic assays to assays for environmental contaminants, biosafety threats, and food diagnostics.
Despite their varying applications, all of these devices share one common element: fluids must be moved to and from various locations within the device in order to work.
Systems for Moving Fluids in the Device
There are two basic strategies for moving fluid in microfluidic devices: “active fluidics” and “passive fluidics.” The active fluidics method moves fluids using active components (e.g., pumps and valves). The passive fluidics method relies on capillary forces to move fluids; it can be enhanced by modifying the internal surfaces of the device with treatments that either accelerate or slow the flow of fluids (see below).
A third, intermediate strategy is to use a centrifugal pump. A centrifugal pump is a device that blends active and passive elements; in this method, surface properties and design features play a bigger role than in a pump-and-valve-based system alone, but must still work together with the centrifugal pump.
Surface Design and Treatment
The surface properties of a microfluidic device’s fluid channels play a significant role in determining how fluid moves through them.
Low surface-energy materials are naturally hydrophobic (i.e., they repel fluids) and are best used with active fluidic elements such as pumps and valves. Examples of low surface-energy materials include Teflon, polystyrene, and polyethylene.
High surface-energy materials are naturally hydrophilic (i.e., they attract fluids) and facilitate fluid movement via capillary action. Nylon, polyesters, polyimides, polyvinylpyrrolidones, and acrylic polymers are examples of high surface-energy materials that can be used to fabricate devices that rely on passive fluidic movement.
Surfaces can be treated with gaseous or liquid reagents to alter their affinities for (or against) aqueous solutions. For example, detergents are reagents that contain both hydrophobic and hydrophilic elements; they can alter the surface properties of a polymeric substrate. Sodium dodecyl sulfate (SDS) is a classic example of a detergent: it has a twelve-carbon lipophilic tail attached to a highly polar sulfate group. One of the protons on the sulfate has been replaced by a sodium ion. When an aqueous solution of this salt is exposed to a hydrophobic surface such as polystyrene, the hydrophobic tail interacts with the surface by binding to it. The polar sulfate group on the molecule now provides a new surface, which is hydrophilic.
Know more about our expertise in microfluidic device design and engineering
Microfluidic Device Dimensions
In general, the sample and reagent volumes that the device is intended to process determines the size of the channels and chambers in the device. There is enough glucose in a drop of blood (approximately 30uL) that only a fraction of that volume is needed for the assay. On the other hand, an assay for thyroid stimulating hormone (TSH) might require 200uL of plasma (or 400uL of whole blood) to achieve the desired sensitivity. Clearly, these two analytes require vastly different microfluidic designs as might be expected by their 10 million-fold (or more) differences in analytical goals.
Another aspect to consider in the design of a microfluidics device is the need to keep various fluids moving as “slugs” along the channels. If the size of the channels or chambers in the device is too small or too large, the “slugs” may suffer from segmentation. In passive devices, that will mean the loss of capillary action. In actively pumped devices, an incorrect fluid volume for the channel size can result in overpressure; this can lead to layer sealing failure and loss of reactants.
Microfluidic Chambers, Junctions, Pegs, et. al.
The channels in microfluidic devices make up only part of a device’s geometry. Other features, such as chambers, T or Y junctions, and pegs are sometimes necessary for desired functionality. Chambers can be prefilled with liquid, lyophilized, or freeze-dried reagents. Junctions are used to introduce different fluids into the reaction mixture. Pegs can be fabricated in the channels to produce turbulent flow and resultant mixing of various fluids (e.g., the sample with reagents) and are often located just downstream of junctions.
In order to introduce samples and reagents into the device, communication with the “outside world” is important. This is accomplished via the use of wells in the larger surface of the device or with specialized connection fixtures (e.g., Luers) on the edges.
Light Considerations
If the device’s signal readout involves measuring photons, the device’s optical properties must be considered. Absorbance (the measurement of attenuation) is regularly used. Fluorescence is more sensitive, but it requires getting light into the device and back out again at appropriate angles for both excitation and emitted light to minimize background noise. Chemiluminescence is the most sensitive detection technology, but the necessity for triggering reagents adds complexity to the design.
If the signal needs to be increased, amplification is one way to do it. Depending on the sample volume, it’s possible to incorporate longer optical pathways into the device’s design.
Integrating all of these design features into a coherent whole is a challenge.
Microfluidic Production Materials
Among the many considerations inherent to designing a microfluidic device, it’s easy to overlook a central component: the plastic and the other materials used in the device assembly. It is best to work with vendors who are experienced in producing microfluidic devices and meet ISO requirements for the raw materials used in the fabrication of the devices.
Suppliers of raw materials can make minor changes in the components that device fabricators use in their processes. For example, consider the mold release agents used in injection molding. A “better” mold release agent can speed up the injection molder’s cycle times, enabling more-efficient production at the potential cost of altered function in the device. Such a change could occur, and without careful record keeping, the microfluidic device developer would never know why the device suddenly started to behave very differently than intended.
Incoming raw material specifications are critical to the development of any bioanalytical method. This applies to the physical device itself; a part may look the same, but it may not act the same. Only rigorous testing against retained components that have been previously shown to be functional can ensure functionality and continuity.
There are many different inputs to consider when designing and developing a microfluidic device and working with an experienced partner can significantly shorten the journey from bench to market. We develop and manufacture life science and diagnostic products including IVD and companion diagnostics. We can help you quickly turn your technology into user-friendly, cost-effective, and clinically validated commercial product.